HY-80 high strength steel
January 18,2023
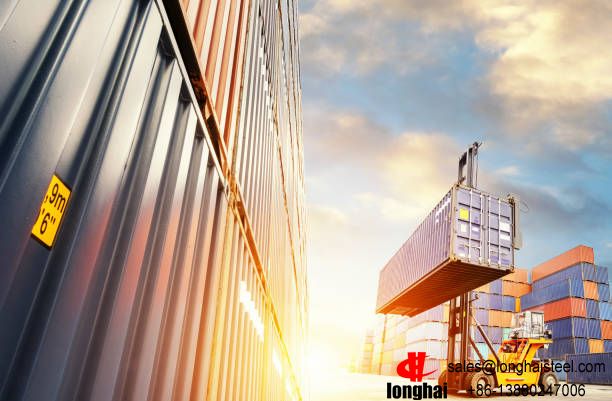
HY-80 is high strength steel used in a quenched and tempered condition to achieve mechanical properties such as ship and submarine hulls, as well as some pressure vessels. This thesis will focus on the HY-80 used in the fabrication of the dive planes on nuclear submarines. Since the material is quenched and tempered, the resulting tempered martensitic microstructures confer the strengthening. However, this leaves the material susceptible to Hydrogen Assisted Cracking (HAC) as described by Masubuchi and Martin. HAC happens when hydrogen diffuses into the material and concentrates at locations such as grain boundaries. This eventually leads to cracking. When conventional fusion welding is used on HY-80, there is a potential for hydrogen to be liberated from the water molecule and to diffuse/dissolve into the fusion zone. Hydrogen diffusion in weld metal cannot be prevented; however, extensive site preparation and strict environmental controls can be used to restrict the amount of diffusible and residual hydrogen in the resulting weldment. The material preparation requires pre- and postheating of the materials to be welded, filler electrode controls such as baking and storing and control of moisture and hydrocarbons. Due to these strict environmental controls, performing conventional fusion welding underwater would require expensive site preparation and, likely, prolonged repair time according to “Underwater repair technology” by Nixon. The material of interest here, HY-80 steel, is high strength steel used in a quenched and tempered condition to achieve mechanical properties desired in many U.S. Navy applications, such as ship and submarine hulls, as well as some pressure vessels. This thesis will focus on the HY-80 used in the fabrication of the dive planes on nuclear submarines. Since the material is quenched and tempered, the resulting tempered martensitic microstructures confer the strengthening. However, this leaves the material susceptible to Hydrogen Assisted Cracking (HAC) as described by Masubuchi and Martin. HAC happens when hydrogen diffuses into the material and concentrates at locations such as grain boundaries. This eventually leads to cracking. When conventional fusion welding is used on HY-80, there is a potential for hydrogen to be liberated from the water molecule and to diffuse/dissolve into the fusion zone. Hydrogen diffusion in weld metal cannot be prevented; however, extensive site preparation and strict environmental controls can be used to restrict the amount of diffusible and residual hydrogen in the resulting weldment. The material preparation requires pre- and postheating of the materials to be welded, filler electrode controls such as baking and storing and control of moisture and hydrocarbons. Due to these strict environmental controls, performing conventional fusion welding underwater would require expensive site preparation and, likely, prolonged repair time according to “Underwater repair technology” by Nixon.
Figure 1. FSW/P Nomenclature [From 4].
In the United States Navy, Los Angeles-class submarines have been found to develop dive plane cracks due to the stresses of changing depth. When found, cracks are noted and scheduled for repair during the next dry docking interval. The repair of the dive planes may entail drilling holes in the dive planes to thoroughly drain out any water and placing of heating blankets. The cracks and these holes are then repaired with conventional fusion welding. This process has the potential to extend the stay in dry dock if problems arise or due to the aforementioned extensive site preparation and strict environmental controls. An alternative to conventional fusion welding is friction stir welding (FSW) and friction stir processing (FSP). FSP and FSW are solid state processes for the joining and processing of different materials. Friction stir welding/processing (FSW/P) were invented by The Welding Institute in Cambridge, United Kingdom in 1991 [3]. FSW/P has been implemented into the U.S. Navy in such applications as joining aluminum material on Littoral Combat Ships along with the surface treatment of nickel aluminum bronze propellers. In the last ten years, research on FSW/P of steels has received increasing attention. The process of friction stirring involves a cylindrical tool with a shoulder and a projecting pin. The tool is spun at pre-determined revolutions per minute (RPM) and then plunged into the material and traversed across it. Friction stir processing can be done on a single sheet of material to change the mechanical properties by changing the microstructure. For friction stir welding, plates can be abutted and joined by traversing the tool along the seam line. An anvil is placed under the material to react the plunge force. As the tool traverses along the weld line, the material undergoes severe plastic deformation under adiabatic conditions and is simultaneously heated via frictional and adiabatic dissipation. Figure 1 illustrates the basic FSW/P nomenclature.
This thesis continues and builds upon prior work conducted by Norman Overfield [5] and William Stewart [6]. Overfield demonstrated that FSW/P can be performed on a hardenable alloy steel (AISI 4142) in dry ambient conditions and also underwater. He also concluded that the hydrogen concentration in FSW/P material for both dry and underwater conditions was well within acceptable levels for high-strength steels. Stewart conducted initial experimentation on FSW/P of HY-80 or 100 both in dry and wet conditions. This thesis verifies that FSW/P can be successfully conducted on HY-80 under both a dry ambient condition and underwater while minimizing defects and redistributing inclusions, along with producing acceptable hydrogen content. Friction Stir Welding (FSW) has been used primarily for joining of low melting metals such as Aluminum and Magnesium alloys. Peak process temperatures are typically 450–500 °C, and conventional tool steels have adequate wear resistance to withstand such operating conditions. However, the application of FSW to steels and other higher melting temperature materials has been limited by the lack of suitable tool materials, since operating temperatures higher than 1000 °C are necessary in order to achieve adequate deformation of the materials to be joined [7]. In recent years, there has been an increase in potential tool materials. DP980 (advanced high-strength steel) [8] and SKD61 tool steel [9] have been examined. However, for steels, polycrystalline cubic boron nitride (PCBN) is the most suitable tool material and was used in this work. PCBN is typically used as an abrasive and is most commonly used as a tool material for iron, nickel and other alloys at high temperature. When PCBN comes in contact with oxygen at high temperatures, a passivation layer of boron oxide forms on the surface and protects the material from further deterioration. [10] After FSW/P, the resulting microstructures are highly dependent upon the chemical composition of the base material as well as the weld parameters; revolutions per minute (RPM), inches per minute (IPM), and plunge force. Parameters ranging from 1000 RPM/ 15 mm/s (35 IPM) [11] to 100 RPM 25 mm/min (1 IPM) [12] have been shown to produce defect-free welds. Recorded plunge force can range from 5 kN (1125 lbf) to 40 kN (8900 lbf) [13]. According to Overfield [3], the best combinations of RPM and IPM for steels were 400 and 2, respectively. This was shown to produce a homogenous and defect-free weld. The plunge force is typically set at a constant known parameter that produces an even weld depth, minimizing the flash. Variable plunge force can be set to compensate with any unexpected changes in material height or surface anomalies. In friction stir processed steels, a martensitic microstructure is commonly produced in the stir zone and the thermomechanically affected zone (TMAZ) [7, 14]. This indicates that the peak temperature in the stir zone is in the austenite range for the material. After rapid cooling, the prior austenite grains become untempered martensite. However, one study was conducted on high strength steel in which the welding parameters and cooling rates were controlled to produce a martensite-free weld by not allowing the temperature in the SZ to not exceed the A1 temperature [14]. This study shows that by controlling the cooling curves, the microstructure can be controlled and therefore the material can be processed to possess different mechanical properties [7, 14]. The only known studies of FSW/P of steel being conducted underwater are those reported by Overfield [3] and Stewart [4]. FSW/P conducted underwater on steels has the potential to replace conventional fusion welding. Underwater fusion welding requires extensive site preparation and strict environmental controls. There is a higher potential for HAC due to the high temperature of the arc under the water. The energy from the arc tends to dissociate the water molecule to produce large amounts of monoatomic hydrogen which then will diffuse into the weld. There are no industry standards to weld HY-80 underwater [15]. HY-80 is a low carbon alloy steel that acquires its strength and toughness through quenching and tempering. HY-80 has an upper limit of 0.18 wt% carbon. Nickel and chromium are used to reduce the ductile-brittle transition temperature and enhance the hardenability. The sulfur and phosphorous contents are strictly controlled so they do not exceed 0.045 wt% together [16]. The composition limits can be seen in Table 1. HY-80 is made by either the open hearth or the electric furnace process. The resulting steel is fully killed and fine grained. Plates are then rolled directly from either ingots or slabs. A final heat treatment of quenching and tempering is conducted to achieve the desired strength and toughness. Due to this final heat treatment, the steel is susceptible to hydrogen assisted cracking which conventional fusion welding can contribute. The phase diagram of Fe-C shows the phases that are produced when performing conventional fusion welding and that of FSW/P, Figure 2. This thesis will compare the hydrogen content between base metal, conventional fusion welding, and FSW/P to determine if FSW/P will also contribute to HAC.